
Thrust 4: Characterizing Dynamics and Defects
Fraño, Steinmetz, and Pascal will develop in situ assembly protocols specific to protein and hybrid building blocks for a suite of characterization tools, since these light (low-Z) materials may not achieve sufficient contrast for XPCS. For example, the nanoscale spatial distribution of polymers and polymer grafts associated with hybrid building blocks will be characterized using SAXS (van Buuren) and X-ray phase tomography (Fraño). In addition, Fraño will explore neutron spin echo (NSE) spectroscopy—which has been demonstrated as a powerful technique for direct observation of protein machinery and the molecular dynamics of polymer chains in relevant space (1-100 nm) and time (100 ns-10 ps) scales—for probing the fast dynamics of protein and polymer components in our assembly systems, including their conformational and diffusional behavior during the assembly process.
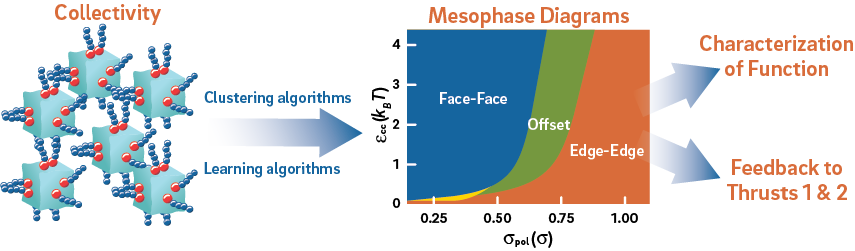
These characterization techniques will provide a powerful means to study both the formation of—and a system’s response to—assembly defects, which are critical for determining mesoscale structure-function. Viral capsid proteins, which readily assemble under a variety of experimental conditions, will provide a unique system of study for defect mitigation. Nature’s ability to design robust assembly processes provides a biological inspiration for designing similarly robust assembly processes in our synthetic materials systems. Using tools developed by Steinmetz for supramolecular capsid assembly, we will study defect formation using genetically programmed electrostatic interactions in two systems: the icosahedral cowpea chlorotic mottle virus (CCMV) and tubular tobacco mosaic virus (TMV). The engineering design space of these systems is paralleled: for example, TMV coat proteins—typically assembled as 300x18 nm tubes made from 2,130 identical coat protein copies—can be shifted into spherical nanoparticles through heat or programmed with specific aspect-ratios using RNA polymer templates. Steinmetz will work with Fraño to experimentally observe how the systematic introduction of point defects to coat proteins using genetic modification or residue-specific chemical modification affects assembly. This will include measurement of assembly kinetics and identification of structural outcomes (e.g., vacancies, rearrangements, or frustrated geometries) in the final protein assembly.
A suite of complementary, experimental characterization techniques (SAXS, Raman, and NSE) will be used to provide multi-resolution details into the structure and dynamics of our assemblies. Each of these techniques leads to spectra that are complicated by both local environment and larger-scale conformational dynamical effects. Precise interpretation of these spectra is therefore crucial for reconciling the atomic, nano, and mesoscale structures that give rise to specific spectral features, and added complexity arises when looking at time-resolved spectra. The Pascal group has recently developed computational tools for simulating spectroscopies at various length (energy) scales115, 116 from microwaves to X-rays, all within a general framework. For example, in a study of a prototypical nanofluidic system, the thermodynamic driving force behind water intercalation into carbon nanotubes was shown to result from enhanced rotational dynamics next to the hydrophobic interface, visible in the microwave regime. In another study of energy storage materials, the dissolution of broken-chain sulfur species into organic electrolytes was shown to result from enhanced vibrational states visible in the IR regime. Pascal will extend this spectrochemistry computational platform to simulate Raman, NSE and XPCS spectra. In particular, Pascal is currently developing methods to incorporate nuclear spin into MD simulations by means of coupled Ising-models. Working with Tao, Fraño and Steinmetz, Pascal will validate this spectral simulator on a variety of model systems, ranging from “isolated” (i.e., low-density assemblies) of bare and grafted NCs from Tao, to the viral TMV assemblies from Steinmetz. Once validated, the spectral signatures of mesostructures predicted from the MD simulations of Arya and Paesani will be computed and compared to experimental data. We will create a self-consistent feedback loop, in which discrepancies in the peak positions and line shapes will be used to further refine the energy landscapes and interaction potentials of the CG models.